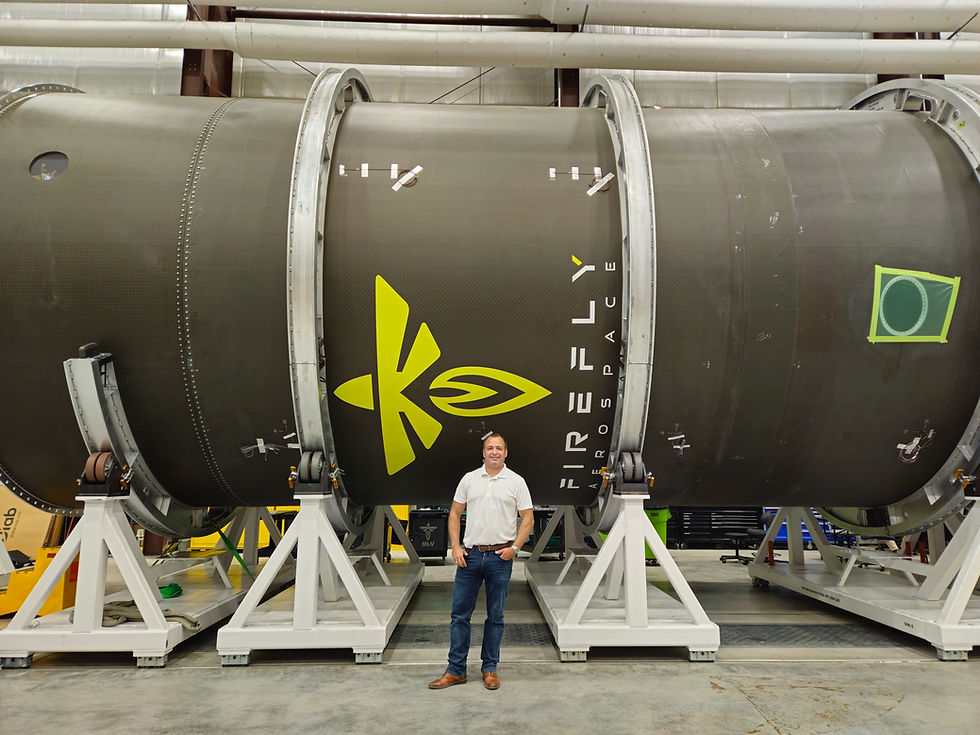
This is my story, about how a kid with the "Knack" that wanted to work on cars, sent something to space, the moon, and is now taking on Aerospace.
I started my technical career as a composite technologist—not necessarily by choice, but because life led me there. Growing up, I looked up to my father, my everyday hero. He occasionally took me to work, where I got to see fascinating "things" and applications firsthand. Even as a kid, I was immersed in the world of adhesives—polyolefins, bumper glues, windshield adhesives, and expanding polyurethane foams used for structural stiffening.
I heard stories about Lotus cars being bonded together instead of welded for weight savings, truck beds assembled in three pieces using adhesives, and windshields popping out due to changes in cure schedules. I even brought intake manifolds from supercars to show-and-tell and somehow convinced a supercar company to send me a rocker panel from what I still consider one of the most beautiful supercars ever built.
Many people helped me along the way, but in some ways, I was helping too—without even realizing it. Some might recognize this as the "knack," as depicted in the famous Dilbert clip, or simply as an insatiable curiosity about how mechanical systems work. For me, it was less about the obvious mechanics and more about the unexpected behaviors of materials and structures. Pair that with boundless energy and a touch of ADD, and it made for an exciting journey.
For those who know me, parts of my story may sound familiar, but I want to share how I got to where I am today and why I believe Angels Aircraft isn't just possible—it’s necessary.
My Story
I grew up as the second youngest of five kids. My mother started as an elementary school teacher, but once the youngest of us came along, she dedicated herself full-time to wrangling our chaotic household. My father, a chemical engineer, climbed the ranks at Dow Chemical.
Growing up I moved frequently— always staying somewhere at least four years, never for more than eight years—but I gained a deep appreciation for the geographic diversity of the U.S. I was born in Louisiana, lived in Baton Rouge while my dad worked with polyolefins, and then moved to New Jersey when I was eight for his new role at Dow’s Essex Specialty Products division, focused on adhesives.
I still remember the day my dad came home and told my mom they had already forecasted the plant closing—before we had even fully unpacked. Despite that uncertainty, New Jersey was a great place to grow up. School had "beach days", we went deep-sea fishing, and my mother, an avid sailor in her youth, made sure my brother and I spent summers sailing at the Manasquan River Inlet.
This was also when I first saw a chemical lab environment. I watched mechanical testing in action and heard about the finicky nature of polymeric adhesives—how they had to cure under exact conditions to function correctly. Most failures weren’t due to poor material performance but rather variations in raw materials or manufacturing inconsistencies. The biggest stories always seemed to revolve around windshield adhesives and manufacturers’ changes in process such as paint and primer changes, accelerated curing with heat and moisture and overall variation of the process both intentional and unintentional. The takeaway? If you follow the instructions, you get predictable results and most things done happen just once, its likely going to happen again.
After four years in New Jersey, we moved to Michigan. For me, the first place that truly feels like "home" is where you learn to drive, and Michigan was that place. It was also where I started putting the pieces together for my future. My dad’s work at Dow Automotive was much closer, giving me even more exposure to cutting-edge materials and manufacturing techniques.
I got to hear firsthand about bonded intake manifolds for Maserati, seven-axis glue machines running parts straight through the tool, Mustang Cobra R projects, and polymeric truck beds being assembled, making parts without warping—like the Avalanche tailgate with its raised lettering. At the time, I was convinced my future was in polymer materials engineering, specifically in the automotive industry, which seemed to have the most exciting tech deployment.
Outside of academics, I played soccer in the fall and helped launch the first FIRST Robotics team at Notre Dame Prep during Junior year. Initially, I wasn’t on the build team but contributed to communications and marketing, helping with branding as we partnered with Avondale High School's Team 33. One of my personal contributions was the hand-drawn "33" logo—still in use today, thanks in part to John Whitmer. By the second year, we made it to the national finals after I made it on the build team of course 😉.
Between Boy Scouts and robotics, I firmly believe in the importance of surrounding young minds with sharp like-minded individuals who can turn ideas into reality. Kudos to Jim Zondag and Tim Grogan, who continue to inspire future engineers through FIRST Team 33.
I always sought challenges, pushing myself with rigorous coursework. My parents didnt allow study halls in high school, so I took every computer science class available and loaded up on AP science and math courses—everything except biology and geology. During course registration, the principal called me into his office and warned me that taking varsity soccer, FIRST Robotics, and five AP classes would be "academic suicide"—and that I wasn’t the kind of student who could handle it. I took that as a direct challenge, ended our conversation with “watch me” and walked out.
Most people understand the demands of varsity sports, but robotics added another layer of intensity. During the six-week build season, we spent four hours after school every day, all day on Saturdays, with one allowed day off per week. After the build, we traveled to three regional tournaments and the national finals in Orlando. I ended my last semester with all A’s and one B (Calculus 4 got me), proving that challenge only fuels determination.
It was time for my college decision. I had applied to a number of top engineering schools and had to decide between University of Michigan and Purdue University. While rankings placed them neck-and-neck, Purdue’s Materials Engineering program was ranked #3 in the nation. I was also concerned about U of M’s downtown campus, knowing my ADD would make it difficult to focus. In contrast, Purdue, surrounded by cornfields, seemed ideal for minimizing distractions. Off to West Lafayette, Indiana.
Purdue was a roller coaster. Initially, I struggled, but as I learned more about materials engineering, I discovered it had three branches: ceramics, metallics, and polymers. Enthusiastically, I chose polymers—only to be told that while Purdue excelled in metallics and ceramics, its polymer program was limited to nearly non-existent. Determined to follow my passion, I pursued a dual degree in Chemical Engineering to feed the need for polymer science.
Balancing the demands of two majors was as challenging as expected. I had to take two Organic Chemistries, two Thermodynamics classes (in which all but 4 or 5 variables changed letters), two Physical Chemistries and the harder of whichever classes overlapped. My typical class load was 21-23 credit hours per semester. In the summers, I general electives and retook math classes if I wasn’t happy with the grade, transferring them as “credit only” to maintain progress. While I was interested in learning new content at the conceptual level, I felt like this was all wrong. I was learning about materials that I didn’t want to and the extent of polymers was labs mixing clear goos together for weeks at a time just to have a different white chunk at the end. At one point, I seriously questioned whether I wanted a career of such monotony—until two professors changed my outlook.
While taking Professor Kvam’s Electrical Properties of Materials course he pulled me aside and told me it seemed like I was applying ADD to my future and that I should focus. Meeting with him weekly helped me focus my energies. This led to one of my focal efforts of my college career into P-N junctions, solid-state energy conversion and photovoltaics. I still have several patent ideas and sealed envelopes that I will try to develop when the time is right. Another professor introduced me to composites, a field I knew little about. By chance, I heard that Dr. Pipes, a new professor with extensive experience in composites, was joining Purdue.
As a junior in materials engineering and a sophomore in chemical engineering, I knew very little about composites. So, I had to do some research. I quickly realized they were about 40% polymer, which piqued my interest. Through conversations with others, I learned that the professor teaching the course wasn’t initially planning to take on students. He had an impressive background—formerly the President of Rensselaer Polytechnic and the founder of the Center for Composites at the University of Delaware. He had been brought in to help other professors make the kind of impact he had achieved in his career. I knew I had to approach him carefully if I wanted a chance to enroll in his class.
I spent far too long thinking about what I would say, but when the time came, I don’t think he saw it coming. I practically begged and pleaded to get into his first course, Manufacturing of Advanced Composites. To my surprise, he let me in as a junior, which inadvertently opened the class to undergraduates as a technical elective. He had expected only 14 graduate students—plus me—but on the first day, 35 students showed up. He had to warn the undergraduates that he didn’t think they would succeed due to the heavy time commitment. He gave one of the most intimidating presentations of his syllabus I had encountered in my college career, most of the undergraduates didn’t come back the next day.
Beyond learning the mathematics behind the models that drive composite manufacturing, the course included a semester-long reverse engineering project. We had to take an industry product and work backward to determine how it was made. At the time, I was already carrying a 21–23 credit course load, and this class alone was expected to take up half my time. It was easily the hardest challenge I had faced so far. What could go wrong? So, I got to work.
One of the coolest things about composites and composite engineering is that most students studying them come from different backgrounds. The class was filled with sports enthusiasts who knew about composites from skiing and snowboarding, pilots who were familiar with them from aircraft, and a third group—where I fell—who knew about them from cars and the automotive industry.
When I was assigned to source a composite product from industry, I started calling companies. I figured the easiest ones to reach would be Wilson for tennis rackets and Burton for ski materials. Then, I decided to take a shot at Ferrari, Lamborghini, and Saleen. To my surprise, none of them responded—except for Saleen. Not only did they provide materials for my project, but they also told me they had funding for a study comparing two different manufacturing techniques used in their UK- and US-produced composites.
Being completely naive, I told them we didn’t need any funding—I was already paying tuition, and my classmate and I would take on the challenge ourselves. Saleen then proceeded to send us some incredible car parts from the Saleen S7. Excited, I convinced my lab partner that we could handle this and reached out to Professor Pipes to get his approval for reverse-engineering car parts. He asked me to bring in a part to examine, then invited me to lunch afterward.
On our way to lunch, we made an unexpected stop at Walmart. He said he needed to pick up a couple of things but didn’t tell me what. I followed him through the aisles, wondering what he was looking for. After a few minutes, he grabbed a hockey stick and a fishing pole. Then, he turned to me and asked if I knew why he was buying them. I assumed it was for a future lecture, but he shook his head. “No,” he said. “These are for the other teams’ projects.”
A few weeks later, Dr. Pipes asked me to come to his office and asked if I had ever considered doing directed research over the summer. He then offered me a job adapting a course he had taught in the 1970s—Experimental Characterization of Composites—to Purdue’s capabilities. Handing me his book, he tasked me with breaking it down into labs that could be carried out based on its content.
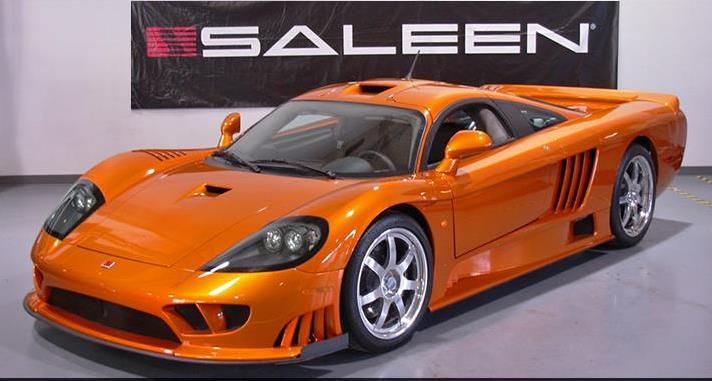
Being as future-focused as I am, I immediately started reaching out to companies to secure the necessary technology and resources. I managed to get donations of composite materials, computers, strain gauge scanners, and strain gauges all at no cost. By the time I proposed the lab structure, I had also brought in $40,000 worth of materials and equipment—because no one had told me there was a budget, I had assumed it was zero.
This marked the beginning of my deep dive into production implementation albeit on a small scale. I had the theory, some coarse instructions, and the equipment—all I had to do was execute and produce roughly 1000 coupons. That semester, I fell into a cycle: after classes, I would build the test coupons during the week, and every Tuesday—the day before class—I would run the lab myself to ensure the data came out correctly and matched the models. Nearly every Tuesday night, I pulled an all-nighter to get things right, and I only delayed the lab once. This meant learning and modifying software, running more Mathematica equations and data than I had ever encountered, troubleshooting mismatched units and decimals, and fixing test setups that weren’t properly aligned. So the class would run smoothly the next day.
One lab, in particular, nearly broke me—the coefficient of expansion of carbon fiber lamina. Thermally cycling the coupons took a long time, and my initial results were completely off. The coefficients were way outside the expected range and had the opposite slope they should have. After nearly two days without sleep, I finally realized that if you cycled the coupon enough times, all the wild variations were just hysteresis. After about 10 cycles of two-hour thermal tests, the expected near-zero CTE curve finally emerged.
During this time, Dr. Pipes started inviting me to research collaboration meetings. For manufacturing-related meetings, we would discuss the problem in advance, brainstorm potential causes and approaches. I would then conduct conceptual tests overnight and present our findings the next day to a very surprised potential customer. This continued through my senior year, when we ran the experimental characterization course again—but this time with a twist.
A graduate student had taken issue with the fact that I, an undergraduate, was leading the lab portion of a graduate-level class. As a result, I was no longer allowed to run the labs. Instead, I pivoted—rather than running the lab myself, I spent the day before each class teaching another graduate student how to do it. A few days before the course started, I was called into a meeting where I was told, “Since you’re not running the lab this time, why don’t you just take the class yourself?” And that’s how I ended up taking my own lab course.
By this point, I wanted to go to grad school but didn’t have the GPA. However, thanks to the publications from my work, I had caught the attention of Boeing and was recruited to work on the 737 Max in their M&P division. At the time, the plan was for it to be a “mini 787” made primarily of composites. I was going to take the job offer but decided to ask Dr. Pipes if he thought it was a good move. That conversation changed everything—I ended up staying at Purdue as a full-time composite manufacturing engineer while enrolling in a full-time master’s program in the evenings.
In this expanded role, I represented the Center for Defense Innovation at Purdue, supporting various professors and research initiatives. I also took on the role of running conference spaces and coordinating projects to ensure their successful execution. We worked on some wild projects—developing vibro-mechanical NDI techniques that used music vibrations to verify structural integrity, experimenting with flash thermography, embedding antennas inside composites, and doing some early work on phased-array antennas.
Entering the Aerospace Industry
Somewhere along the way, at a conference, I presented to an individual from Lockheed Martin Skunk Works. I don’t remember having much of a conversation with him, but my advisor had encouraged me to hand out résumés to get a sense of my market value.
I had known about Lockheed Martin Skunk Works since I was about five years old because my older brother was obsessed with the SR-71, stealth fighters, and space. He talked about them constantly. At the time, I considered myself an average student and a pretty ordinary person, so I saw a job at Skunk Works as a “Hallelujah” position—something only the top of the class would land. It was such a high-demand job that I never would have had the confidence to apply on my own.
But then I got a phone call. A recruiter from Lockheed Martin Skunk Works reached out, saying, “We have a job for you. We’d really like you to come out to Palmdale and see if you think it would be a good fit.” And that’s how I ended up at Lockheed Martin, stepping into the aerospace industry. Life took me to Palmdale California next.
I worked in AMS&P (advanced manufacturing and prototyping), developing cutting-edge technologies and refining them until they were mature enough to transition onto active programs. A lot of our work centered around digital factories, digital threads, and structural optimizations, but a big part of the job was scaling up technology to operational sizes and testing whether the original promise still held true. This experience solidified a fundamental approach that I’ve carried throughout my career: hands-on execution is far more valuable than a literature search. Research is useful when you have a well-defined problem and a clear path forward, but if you go and build a development concept, you’ll find that roughly 85% of the solution falls into place naturally. The real development effort should then focus on that last 15%—the parts that aren’t working as expected. This is a far more efficient way to innovate.
During my time at Lockheed Martin, I ruffled a lot of feathers and stepped on more than a few toes. I had come from an environment managing an eight-figure annual budget to a division where managers, senior managers, engineers, and technicians were all working under a seven-figure budget. It was frustrating to propose well-founded solutions, only to be dismissed by people who didn’t fully understand the problem—especially when we were actively pursuing solutions that we knew didn’t work.
Despite the challenges, I was still able to make meaningful contributions. I helped increase capabilities in key areas and worked on developing tools that, frankly, the company wasn’t quite ready for. However, when the government went through sequestration in 2013, I was let go. The reasoning was blunt: “We know all the new programs we’ll have for the next five years. You need to find your way onto one of those programs because we’re not going to continue funding work for a program that won’t fly.”
Through it all, I had an excellent mentor—Glenn Weissinger, the Vice President of Strategic Planning. He helped me navigate the complexities of Lockheed Martin’s structure, trying to make sense of my objectives and how to collaborate effectively. Coming from Purdue, the culture shift was stark—the average age in Palmdale at the time was 53, and for my one-year anniversary, the company gave me a book titled Love It, Don’t Leave It. Glenn shared invaluable insights, but one piece of advice resonated the most: “You need to get out of Palmdale. Not because you can’t contribute, but because in a classified environment, no one will ever hear about your contributions.” That advice shaped my next career move.
So, I had a choice: join United Technologies to work on classified programs or help L3’s ESSCO division establish a new product line for airborne radomes. I chose radomes and electromagnetic transmissive structures. This stage of my career contrasted sharply with my previous experience. At Lockheed, I had been too aggressive, stepping on toes. Now, I entered this much more cautiously.
At L3 ESSCO in Ayer Massachusetts, I had a strong advocate in my hiring manager, Richard Hitchcock. In my first week, I was called into a senior staff meeting with the president and vice presidents. Richard introduced me as a business development solution for the airborne market. The president, in a thick Bostonian/New York accent, asked bluntly, “What are we doing?” I gave a politically correct answer about needing time to understand the team and past efforts. His response? “The plan was to hire you. What are we doing?”
This was the opposite of my experience at Lockheed. They wanted me to run as fast as I could—so we ran. I reached out to industry connections, explored airborne systems for F-15s, F-16s, Apache helicopters, and Fire Scout drones, and even remanufactured the E2D Hawkeye downward-looking radome. We engaged with major players in the commercial aircraft satellite communications market and emerging flat-panel phased array technologies.
As our programs expanded, we needed more engineering support, so I became the engineering manager for these new products. Once designs were completed, I also had to establish the manufacturing line for production. Beyond airborne platforms, I worked on unique projects—such as designing an 100-meter neutrally buoyant metal space frame radome to be built 1,000 feet underground, watertight to separate ionized water from dirty water for a neutrino collector.
Another major breakthrough was developing linear-oriented thermoplastic composites. The inspiration came from a customer visit at my previous employer, where I saw them layering fuzzy material sheets, in a multi panel compression system, compressing them under heat, and producing dragon-scale ballistic armor plates. At L3, my focus was electromagnetic transmissive structures, and I realized that the fibers in our composites—E-glass, S-glass, and Astroquartz—had relatively poor electrical properties. The best fiber was worse than the poorest resin. If we wanted better radomes, we needed to eliminate the fiber.
I reached out to my former supplier and requested a panel of their dragon scale material. When they asked what thickness, I had no answer, so I just said, “Bulletproof.” During testing, the material’s impact on electrical properties was so low that the noise variation in our measurements was higher than the actual absorption. Instead of radomes absorbing 30-50% of the energy, this new material only absorbed 2%. We patented the technology, not just for its material advantages but for the novel manufacturing techniques we discovered. The best part? It had broader frequency ranges, allowing more capability, it was bulletproof, and it was significantly more affordable than the part it replaced.

That’s how I became a Technical Fellow for Composite Materials and Processes in my 30s, focusing on materials, manufacturing techniques, sales engineering, and operations.
I almost had the opportunity to lead composites production at SpaceX. I flew out for an interview—one of the most intimidating experiences of my career. We started the interview next to the first Falcon 9 that landed, then walked under the unmanned crew capsule, then walked the production area it was massive, mostly metal and very loud. The scale of SpaceX is impressive, and it is very difficult to follow up a plant tour with why you are the right person to lead a significant portion of it. Ultimately, I wasn’t offered the position. Looking back, I later learned from people who were in the room that SpaceX decided to switch the BFR’s structure from composites to stainless steel less than a month later. I’ll never know if that decision was made because I wasn’t there to champion composites with an obvious development path, or if it would have happened regardless of my presence. Either way, it was a challenge I’m not sure I was ready for at the time.
Three months after my interview at SpaceX, I got a call from Firefly Aerospace. At the time, I was still working at L3, where I had created a lot of value, but I had reached a point where I needed a competing offer to negotiate better compensation. This put me in a tough moral position. As an engineer, I tend to see the world in black and white—you’re either all in or you’re not. But in this case, I had to be all in on two opposing opportunities. After talking it over with my wife, she encouraged me to at least go and listen.
What intrigued me about Firefly wasn’t just that they were using composites—it was their audacious goal: to build the largest liquid-fueled composite rocket ever to fly. Many companies had attempted this and failed—even SpaceX had abandoned the approach—so much of the industry believed Firefly was destined to fail as well.
I remember telling Tom Markusic, “I’m confident I can build the parts. I don’t have direct experience in cryogenic composites, but I do have experience in production implementation and was part of the team that brought automated fiber placement online—which we’ll need to scale production.” He then asked if I was intimidated by having to manufacture 30-foot-long composite structures. Having worked on even larger composite wing skins, I wasn’t fazed.
So I said, “Let’s Do this!”
In January 2019, I moved to Austin, Texas, to become Director of Structures at Firefly Aerospace. My team was young—the average age was in the 20s—and youthful exuberance was an understatement. I came into an established company with an established culture that had developed a lot of capability and knowledge. Through circumstance they even had the fortune of learning from their first iteration in the Firefly space systems vehicle and have the opportunity to take everything they learned and reimagine and create the next iteration. The culture that they brought was night and day against the establishment and the companies that I previously worked for. Space had some guided regulations but we were the first to build an all composite liquid fuel vehicle and tend to fly out of a US range. That means we had to prove that the vehicle was capable of what we said it was. I started out too conservative and tried to use a building block approach. I got shot down pretty spectacularly getting told I was hired to build a rocket, not a little rocket, not part of a rocket but the whole rocket. Now go build a rocket!
We focused on building strong relationships within the established aerospace market, partnering with vendors who were willing to support our path to flight—knowing that our success would ultimately bring them profitable customers.
Qualifying a launch vehicle requires building multiple rockets, not just one. This includes development and prototype models, a structural qualification vehicle, a stage qualification vehicle, and the final flight vehicle. If manufacturing processes are immature or material properties are not well understood, even more builds may be necessary. At early Firefly, a common saying captured this iterative process: “Make it, break it, make it, break it, make it, fly it.” And that’s exactly what we did. While we had approximate material data, it lacked the specificity of material allowables, which are tied to actual manufacturing processes and production teams. This data helped us identify failure mechanisms and relative strengths, but I knew we needed a more structured approach.
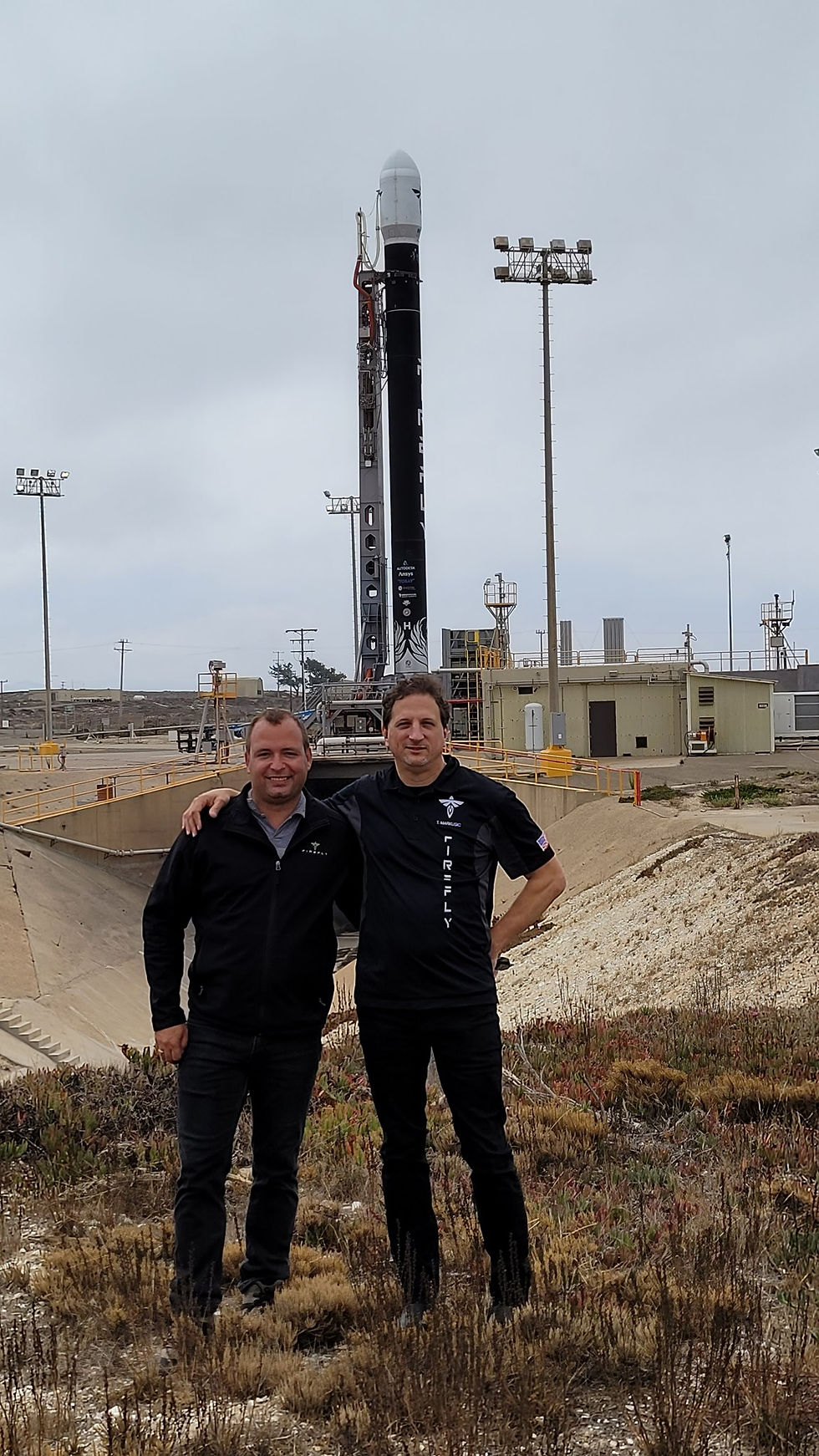
To address this, we worked closely with our suppliers to develop a methodology based on aerospace material allowables, similar to those used by the FAA. This enabled us to accurately characterize our material systems, create a digital twin, and develop a material simulation model for integration into Finite Element Method (FEM) software. We insourced much of this work, and I led the creation of the Materials & Processes (M&P) team, responsible for characterizing reactive chemistries, maintaining strict control over the procedures governing those chemistries, and troubleshooting any material-related issues.
We adopted and refined aerospace composite methodologies, integrating them into our rocket development process. Within my first two years at Firefly, I believe we had successfully defined what we wanted to build and how to achieve it.
The next phase of my journey involved taking on LEAN manufacturing and adapting the principles I had implemented in previous companies to suit a highly rapid manufacturing environment. This was a significant challenge because, in many ways, our senior technicians were acting as unofficial manufacturing engineers. Anyone with extensive experience in composites knows that it is a blend of both design and art—design defines what you want to build, but the process of getting there requires a deep level of craftsmanship.
During this time, I watched three production managers come and go, many of them coming from a metallics background, as Firefly was heavily invested in metallic structures. After some time, I was given the responsibility of optimizing our manufacturing processes specifically for composites. One of the biggest inefficiencies I noticed was unnecessary movement—people constantly walking across the entire facility just to grab a pair of scissors, make a few cuts, and then return the scissors to their original place so no one else could use them.
Another issue was specialty technicians—not because they were hired for specific roles, but because certain parts could only be made by one or two people. To address these inefficiencies, we focused on standardizing processes. We created consistent work instructions that any reasonably trained technician could follow. We also introduced part-specific toolboxes that were shadow-boxed with only the necessary tools, eliminating wasted time searching for equipment.
We then brought in a dedicated team of subject matter expert manufacturing engineers and tasked them with supporting the manufacturing floor. Their primary objectives were to document processes, create repeatable work instructions, and take full ownership of manufacturing workflows to minimize errors. Their goal was to make it as difficult as possible to do things incorrectly. This involved developing detailed work instructions, verification travelers, fixtures, and ensuring that key details and features were locked into place within the broader assembly process.
In manufacturing implementation, there are countless ways to run into problems unless intelligent procedures are paired with baseline training to ensure success. Over time, we became increasingly efficient at building the rocket, systematically reducing variability in the process. As we improved, the focus shifted to scaling production—building not just one rocket, but many. However, we quickly ran into a logistical challenge: limited space. The same floor space used to assemble one rocket was also needed to start the next, but that space was occupied by the vehicle’s final integration. Rockets, by nature, are large, and they can quickly consume all available floor space, forcing us to rethink how we managed production flow.
Our next challenge was envisioning the company’s investment needed to affordably scale rocket production. Building on our manufacturing instructions, workflow analysis, and predicted maximum production rates, we also kept an ear open to potential future products that could shape our operations. Using digital modeling, we designed and optimized multiple iterations of what this future factory might look like.
Composites present a unique challenge in manufacturing. Their fabrication requires an extremely clean environment, yet the trimming and drilling process generates significant debris, creating a dirty workspace. Afterward, components must return to a pristine environment for assembly. Balancing these contrasting conditions was critical in designing an efficient production flow. I’ve discussed this in more detail in a previous blog about production efficiencies and manufacturing floor design.
For the last few years at Firefly, I continued to oversee structures engineering, structures manufacturing, structures manufacturing engineering, and Materials & Processes (M&P). However, a significant portion of my efforts went into ensuring the next-generation Advanced Composite Manufacturing Facility was not only designed correctly but successfully brought to life. I won’t go into further details to avoid treading into intellectual property, but if you ever get the chance to tour the facility in Briggs, Texas, it’s truly incredible to see the vision come to life.
During my five years with Firefly, we built the lunar lander, the OTV, the SUV, the Elytra vehicles, and the machines, fixtures and tools that will produce the MLV. I did not leave Firefly in a void but in the hands of an incredibly capable team. I left to bring this rapid innovation approach to aerospace and aircraft.
And now, tomorrow night (March 2, 2025, at 2:45 AM CST), that lander will touch down on the Moon.
Through all of this, I’ve learned a great deal—and my passion for creating real value has only grown stronger. To me, value comes from expanding capabilities while eliminating inefficiencies. I want to build a team that pushes the boundaries of innovation while embedding efficiency into every process, ensuring that the results are both tangible and impactful.
I chose my next direction as aerospace because I believe it is where I can contribute the most. Aerodynamically optimized structures, designed to move through the wind, are a thing of beauty. More than that, I believe we can design them to be less complex to assemble, easier to meet mission requirements, and far more versatile than what exists today.

Comments